Common planetary misconceptions include believing Earth’s seasons result from distance to the Sun (they’re caused by axial tilt), thinking planetary orbits are perfect circles (they’re elliptical), and assuming the Moon alone controls tides (the Sun contributes 46%). You’ll also find planets don’t maintain constant speeds, with Earth moving fastest at perihelion. These gravitational relationships create fascinating patterns of stability throughout our solar system you’re about to discover.
The Gravity Myth: How Planets “Fall” Around the Sun
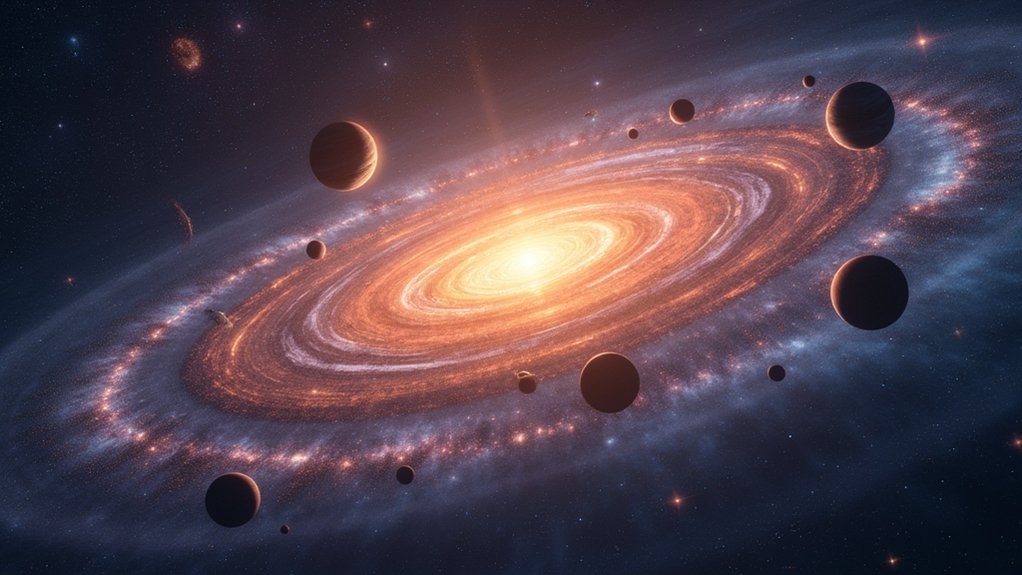
When you look up at the night sky, you’re witnessing an incredible celestial dance—planets in perpetual free-fall around our Sun.
Contrary to popular belief, planets don’t actually fall into the Sun despite its enormous gravitational pull. Instead, they maintain their orbit through a perfect balance between gravity and their tangential speed.
Newton’s law of universal gravitation explains this relationship: the Sun attracts planets while they move fast enough to counteract being pulled inward.
Einstein refined this understanding by describing how massive objects warp space-time, creating curved paths that planets follow naturally.
Your distance from the Sun matters too—Earth races at 67,000 mph to maintain its orbit, while planets at greater distances travel slower but still achieve perfect equilibrium in their cosmic free-fall.
Perfect Circles vs. Reality: The True Shape of Planetary Orbits
For centuries, humans believed planets traveled in perfect circles around the Sun, a tidy concept that aligned with ancient views of celestial perfection. This persistent myth was debunked by Johannes Kepler, who demonstrated planetary orbits are actually elliptical.
You’ll notice this reality when examining Mercury’s orbit with its 0.2056 eccentricity—the most elongated of all planets. This elliptical path means the average distance from the Sun constantly changes, creating varying gravitational pull that affects a planet’s speed.
Earth’s orbit, for example, fluctuates by about 3.1 million miles between perihelion (closest approach) and aphelion (furthest point).
Earth oscillates millions of miles in its elliptical dance around the Sun—the cosmic rhythm shaping our seasons.
These orbital dynamics create complex interactions throughout our solar system, even enabling spacecraft to use gravitational assists during missions—something impossible if planetary paths were perfect circles.
Seasons and Distance: Unraveling Earth’s Position Confusion
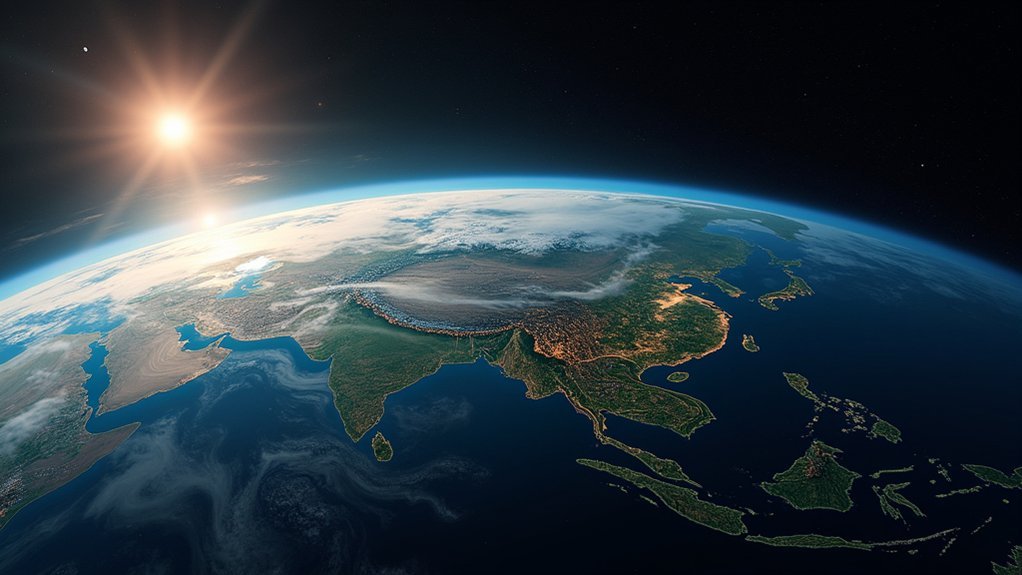
One of the most enduring misconceptions about Earth’s seasons stems from a fundamental misunderstanding of our planet’s position relative to the Sun. Contrary to popular belief, Earth’s distance from the sun doesn’t drive seasonal changes—it’s our 23.5-degree axial tilt that matters.
Seasonal Factor | Northern Hemisphere | Southern Hemisphere |
---|---|---|
Summer timing | July (farthest from sun) | January (closest to sun) |
Cause of warmth | Direct sunlight due to tilt | Direct sunlight due to tilt |
Winter factor | Indirect sunlight | Indirect sunlight |
Temperature driver | Sunlight distribution | Sunlight distribution |
You’ll find it surprising that Earth’s elliptical orbit only varies by 3.1 million miles throughout the year. This minimal distance change can’t explain dramatic seasonal temperature variations, but the changing angle of sunlight distribution across Earth’s surface—caused by axial tilt—perfectly accounts for our seasonal cycles.
Tidal Forces: Beyond Simple Moon-Earth Interactions
Although most people attribute tides solely to the Moon’s influence, this common misconception overlooks the Sun’s substantial contribution, which accounts for nearly half (46%) of Earth’s tidal effects.
The gravitational pull from both celestial bodies creates two daily cycles of high and low tides in most coastal areas.
Three key facts about tidal forces you should know:
- The Moon creates two tidal bulges on Earth—one facing it and another on the opposite side—resulting in high tides approximately every 12 hours.
- Spring tides occur during full and new moons when the Sun and Moon align, creating more extreme tides.
- Neap tides happen during quarter moons when gravitational forces partially cancel each other.
Local geography dramatically impacts tidal ranges, with some regions experiencing variations exceeding 50 feet due to coastal shape and water depth.
Planetary Speeds: Why Closer Doesn’t Always Mean Faster
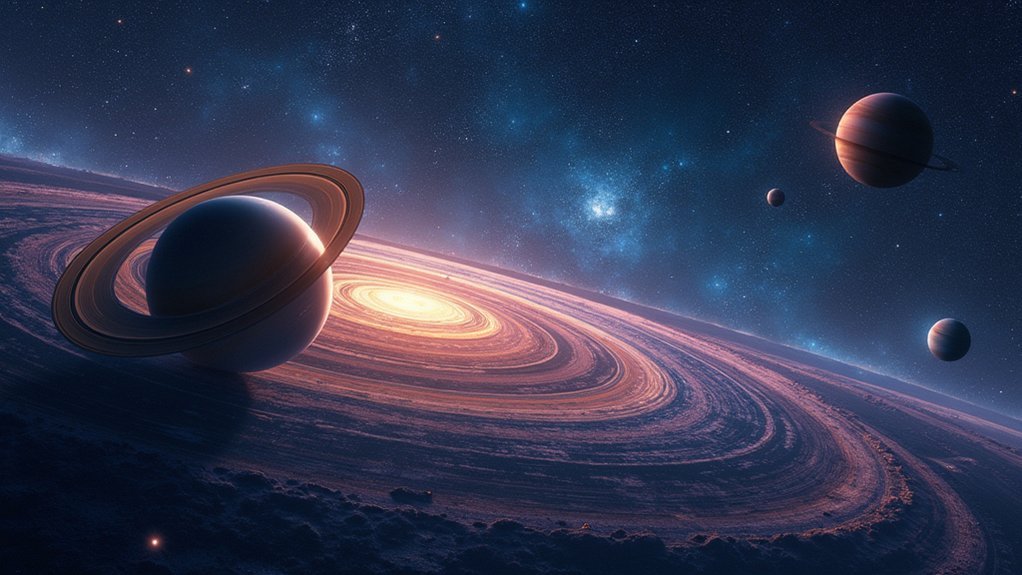
When examining the motion of planets around our Sun, you might assume that planets closer to the Sun always travel faster than those farther away. This assumption is actually correct for orbital speed, but requires proper understanding.
Inner planets like Mercury experience stronger gravitational pull from the Sun, resulting in faster planetary speeds. Mercury orbits at an impressive 47.87 kilometers per second, completing its journey in just 88 days. Earth moves at a moderate 29.78 kilometers per second.
This relationship is precisely described by Kepler’s Third Law, which states that the square of a planet’s orbital period is proportional to the cube of its distance from the Sun.
While outer planets like Jupiter have greater mass and longer orbital periods, their actual speeds are slower due to their greater distance from our star.
Orbital Resonance: The Hidden Rhythms of Solar System Bodies
You’ll find fascinating synchronization patterns throughout our solar system, where planets and moons establish rhythmic orbital relationships that create stability over billions of years.
These resonances form a cosmic dance that prevents collisions and maintains order, with Neptune and Pluto’s 3:2 relationship serving as a prime example of how overlapping orbits can coexist harmoniously.
Beyond simple orbital timing, these gravitational relationships also create stable Lagrangian points where smaller objects can become trapped, forming clusters that remain fixed relative to their larger planetary neighbors.
Planetary Synchronization Patterns
Despite appearing random at first glance, our solar system’s celestial bodies move in remarkably synchronized patterns through orbital resonance. You’ll find these hidden rhythms maintaining cosmic order through gravitational interactions between tidally locked objects. The 2:1 resonance between Jupiter and the asteroid belt prevents those rocks from coalescing into a larger body.
These synchronizations manifest in fascinating ways:
- Neptune’s moons exhibit 3:2 orbital resonance, enhancing their orbital stability.
- Jupiter’s moon Io experiences increased volcanic activity due to resonance with neighboring moons.
- Exoplanetary systems reveal similar patterns, providing critical insights into planetary formation.
When you observe a moon as it orbits its planet, you’re witnessing celestial bodies dancing to gravitational music—a cosmic ballet choreographed by the mathematics of orbital resonance.
Lagrangian Point Stability
Beyond orbital resonance, our solar system contains special regions where gravitational forces create extraordinary stability—these are Lagrangian points.
You’ll find five of these points in the Earth-Moon system where a smaller object can maintain a stable position relative to both bodies. L1 hosts solar observatories like SOHO, allowing continuous sun monitoring.
The most stable points—L4 and L5—sit 60 degrees ahead and behind in orbit, similar to Jupiter’s Trojan asteroids.
Don’t confuse all Lagrangian points as equally stable. L1, L2, and L3 exist in unstable equilibrium, requiring periodic adjustments to maintain position.
Meanwhile, L4 and L5 offer natural stability. These points demonstrate how orbital resonance creates hidden rhythms between celestial bodies, synchronizing their movements and enhancing stability in specific regions of space.
Frequently Asked Questions
What Is the Mythology of Space?
Space mythology includes your misconceptions about perfect planetary alignments, visible planets, the Moon’s “dark side,” crowded asteroid belts, and the Sun’s color. You’ll find these beliefs don’t match astronomical reality.
In Summary
You’ve now seen how common misconceptions about planetary motion don’t match reality. Orbits aren’t perfect circles, Earth’s seasons aren’t caused by distance changes, and tidal forces involve complex interactions. You’ll notice planetary speeds follow specific physical laws, while orbital resonances create fascinating patterns throughout our solar system. Next time you look at the night sky, you’re equipped with accurate knowledge about how these celestial bodies truly move.
Leave a Reply