Declination matters in space navigation because it affects your spacecraft’s orientation relative to both Earth’s magnetic field and celestial coordinates. As you travel from Earth to deep space, you’ll need to shift from magnetic to stellar navigation systems. Even small declination errors can compound dramatically over astronomical distances, potentially causing mission failure. Both historical missions like Apollo and modern Mars rovers have relied on precise declination calculations to guarantee accurate trajectory and positioning. The difference between success and catastrophic failure often lies in these subtle measurements.
Numeric List of Second-Level Headings
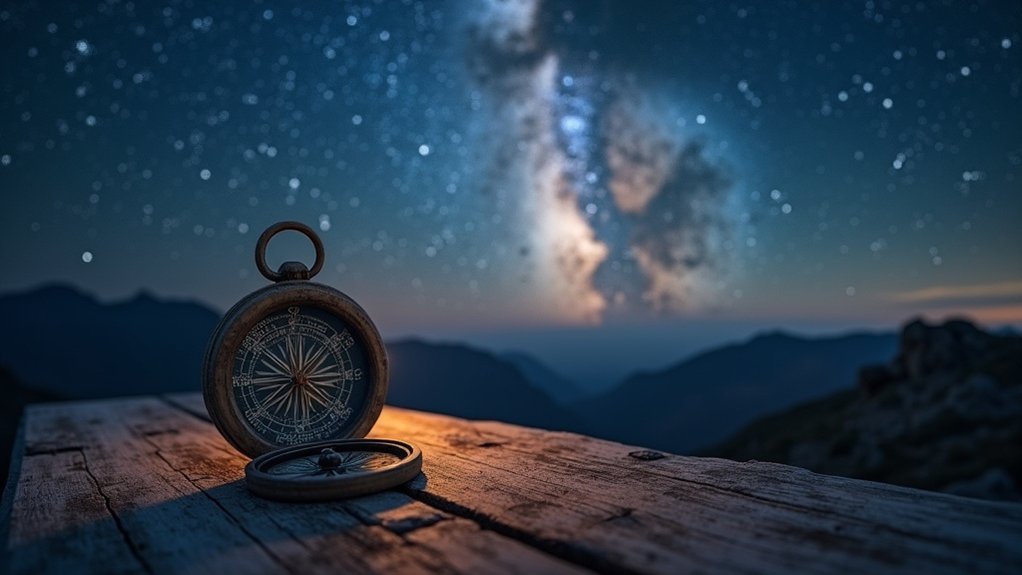
Five key areas comprise our exploration of declination in space navigation. When you’re planning or executing space missions, you’ll need to understand these critical aspects:
- Fundamentals of Magnetic Declination in Spaceflight
- Impact on Launch and Re-entry Calculations
- Long-distance Navigation Precision Challenges
- Real-time Monitoring Systems and Protocols
- Advanced Predictive Models for Declination Compensation
Each section examines how magnetic declination affects different phases of space travel.
You’ll discover why even small declination errors can compound dramatically over astronomical distances, potentially compromising mission success.
The Earth’s magnetic field variations create navigation complexities that require constant adjustment throughout mission planning and execution.
As you’ll see, technologies like the World Magnetic Model provide essential compensation, allowing spacecraft to maintain proper trajectory despite these natural variations in Earth’s magnetic field.
Defining Declination in Celestial Navigation
When you’re steering by the stars, you’ll need to understand declination—the celestial equivalent of Earth’s latitude that measures a star’s angular distance north or south of the celestial equator.
You can visualize declination as ranging from +90° at the north celestial pole to -90° at the south celestial pole, with 0° marking the celestial equator itself.
Your accurate measurement of a star’s declination using tools like a sextant allows you to determine your own latitude at sea, making it a fundamental component of traditional maritime navigation.
Celestial Coordinate Basics
To navigate effectively through the cosmos, you’ll need to understand declination—one of the fundamental coordinates in celestial navigation.
Declination represents the angular distance of a celestial object from the celestial equator, measured in degrees north or degrees south, much like latitude on Earth.
This coordinate is essential for position determination because it helps you calculate how high stars will appear above your horizon based on your location.
When you’re plotting your course through space, declination values allow you to accurately measure altitude and azimuth angles of celestial bodies.
Remember that declination values change over time due to equinox precession, so you’ll need updated star charts for precise navigation.
In the northern hemisphere, Polaris, with its declination near +90 degrees, serves as a reliable reference for finding true north.
Star Position Measurement
Although navigation technology has evolved dramatically, understanding how to measure a star’s position remains fundamental to space navigation.
When you’re tracking celestial bodies, declination serves as your celestial latitude indicator, telling you precisely how far north or south a star sits from the celestial equator where declination is zero.
You’ll measure declination in degrees, with northern positions marked as positive values and southern positions as negative.
Take Polaris, for example—its declination of approximately +89.3° makes it invaluable for northern hemisphere navigation.
To accurately navigate by stars, you must precisely determine declination alongside right ascension.
Together, these coordinates form a reliable positioning system that works even when modern technology fails, allowing you to pinpoint your location using nothing but the stars above.
The Earth-Space Declination Interface
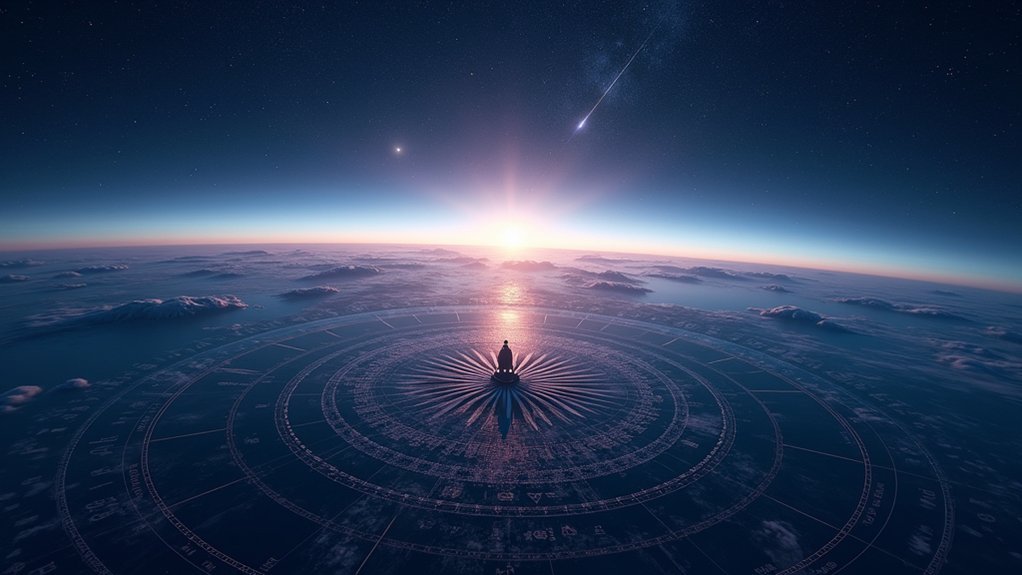
Since spacecraft must navigate between Earth’s magnetic influence and the void of space, they face unique challenges at the Earth-Space declination interface.
As your spacecraft ascends, you’ll need to shift from navigation systems that account for magnetic declination to celestial methods using stars as reference points.
Your onboard sensors are calibrated differently in these environments. Near Earth, magnetic declination affects satellite navigation systems that rely on positioning relative to both magnetic and true north.
However, as you climb higher, Earth’s magnetic field weakens, reducing these effects on your instruments.
The magnetic tether loosens its grip as you ascend, freeing your instruments from Earth’s directional bias.
This shift zone requires careful calibration of your navigational equipment. You’ll need systems designed to function accurately in both domains, automatically adjusting as your spacecraft moves between terrestrial magnetic influence and the celestial orientation requirements of deep space.
Historical Development of Space Declination Calculations
You’ll find fascinating insights when tracing how space declination calculations evolved from early navigators using celestial techniques to the sophisticated systems employed during the Apollo missions.
The earliest orbital missions faced significant challenges as astronauts and engineers grappled with magnetic declination effects on navigational instruments in the unique environment of space.
NASA’s Apollo program marked a pivotal advancement in declination calculation methodology, as engineers developed innovative solutions to compensate for magnetic variations during lunar trajectory planning and execution.
Historical Development of Space Declination Calculations
While terrestrial navigators had long contended with magnetic declination, the birth of the space age in the mid-20th century introduced new complexities to this navigational challenge.
Engineers quickly realized that accurate trajectory planning required precise magnetic declination adjustments, especially during launch and re-entry phases.
A significant breakthrough came in 1996 with the World Magnetic Model (WMM), providing standardized calculations that account for Earth’s constantly changing magnetic field.
This framework, along with historical data from the International Geomagnetic Reference Field (IGRF), enabled mission planners to understand long-term trends in magnetic variation.
Over time, the integration of declination data into navigation software dramatically improved orbital maneuver precision, helping spacecraft avoid potential errors caused by magnetic variations in low Earth orbit.
Early Orbital Navigation Challenges
When the first spacecraft ventured beyond Earth’s atmosphere in the late 1950s, engineers faced unprecedented navigational hurdles related to declination calculations. Without GPS systems, they relied heavily on ground observations and celestial mechanics to determine spacecraft positions.
You’d be surprised how vital magnetic declination was—even small errors could send vessels wildly off course.
Apollo missions revealed that neglecting these calculations could threaten both mission success and astronaut safety. Engineers developed specialized models like the WMM to track Earth’s shifting magnetic field and update navigation algorithms accordingly.
These early challenges demonstrated that inertial guidance systems required continuous adjustment to account for magnetic declination variations across different orbital paths. Even as technology progressed, understanding declination remained fundamental to aligning satellite orbits properly with Earth’s geographic coordinates.
Apollo Mission Adaptations
As spacecraft ventured beyond Earth’s protective magnetosphere during the Apollo era, NASA engineers faced unprecedented challenges in magnetic navigation.
The Apollo Guidance Computer incorporated sophisticated algorithms that accounted for Earth’s magnetic declination variations, vital for maintaining accurate trajectory calculations.
You’ll find it interesting that astronauts underwent specialized training to understand how declination affected their instruments, particularly since traditional compasses became useless in the vacuum of space.
The innovative inertial navigation systems developed for these missions relied heavily on precise declination data to maintain proper orientation throughout the journey.
Mission planning teams meticulously analyzed historical magnetic declination patterns to anticipate navigation issues before they occurred.
These calculations proved essential for safely guiding spacecraft to the Moon and back, ensuring calibration remained ideal even as they traveled beyond Earth’s magnetic influence.
Impact of Declination Errors on Orbital Mechanics
Since spacecraft rely heavily on precise navigational calculations, magnetic declination errors represent a critical vulnerability in orbital mechanics. When you’re operating satellites, even minor declination miscalculations can cause significant positional drift over time.
Your spacecraft’s attitude control systems depend on consistent declination adjustments to maintain proper orientation relative to Earth’s magnetic field.
You’ll notice these errors compound dramatically during long-duration missions, where cumulative deviations can push spacecraft far from their intended paths. This isn’t just a theoretical concern—it directly impacts your mission’s success and safety.
Ground controllers must implement real-time corrections to compensate for declination variations, ensuring satellites stay within designated orbital parameters and avoid potential collisions. Without these adjustments, communication systems and data collection capabilities become increasingly compromised.
Magnetic Fields and Their Effect on Spacecraft Orientation
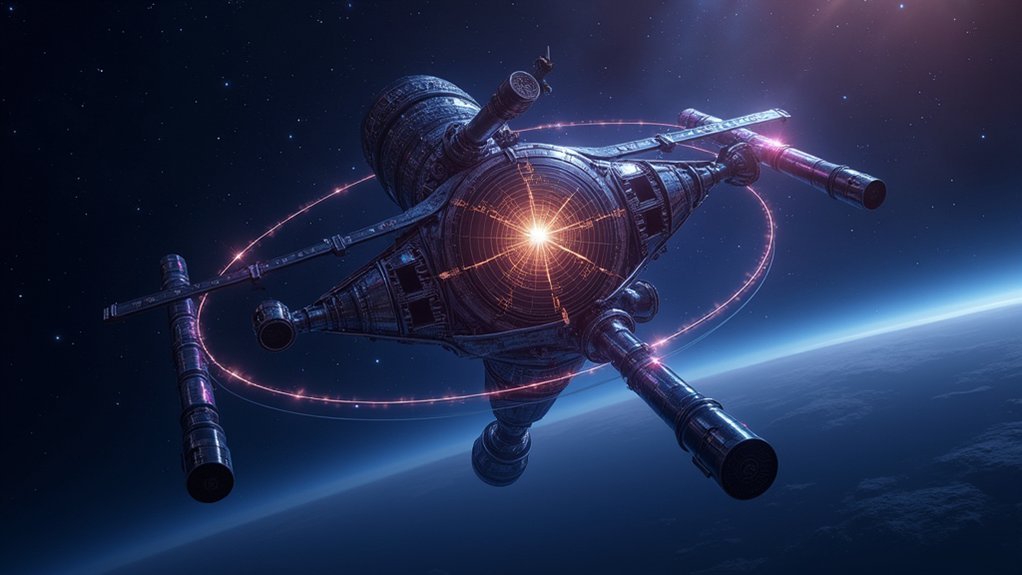
The interaction between magnetic fields and spacecraft represents a fundamental challenge in space navigation. As your vessel orbits Earth, it encounters the planet’s complex magnetic field, which influences trajectory and attitude control. You’ll need magnetometers to measure these fields and determine your orientation relative to the magnetic declination.
Magnetic Field Effect | Impact on Spacecraft |
---|---|
Declination variation | Navigation accuracy loss |
Field strength changes | Attitude control challenges |
Magnetosphere interaction | Communication disruption |
Temporal field shifts | Required algorithm updates |
For low Earth orbit missions, you must account for the discrepancy between magnetic north and true north. Without proper compensation for magnetic declination, your reentry calculations could be compromised. This is especially critical as Earth’s magnetic field gradually changes over time, requiring your navigation systems to incorporate updated declination data throughout extended missions.
Declination Measurement Techniques for Spacecraft
Spacecraft engineers rely on stellar reference systems that compare observed star positions with known celestial coordinates to calculate precise declination measurements.
You’ll find inertial guidance units working alongside these systems, using accelerometers and gyroscopes to continuously track orientation changes even when stellar references aren’t visible.
When calibrating magnetometers for Earth-orbiting missions, you must account for the spacecraft’s own magnetic field, which otherwise introduces measurement errors that compound over long-duration flights.
Stellar Reference Systems
Navigational success in the cosmos depends heavily on stellar reference systems that provide spacecraft with precise orientation and positioning data. When you’re steering billions of miles from Earth, declination measurements become your celestial latitude, allowing your spacecraft to establish its exact position relative to the celestial equator.
These reference systems function through astrometric techniques that precisely measure star positions, creating a steering framework for your vessel. As you travel through space, you’ll rely on this declination data to make critical course corrections and maintain trajectory.
The Deep Space Network utilizes these measurements to track your spacecraft across varying gravitational fields and distances. Advanced celestial models, similar to Earth’s World Magnetic Model, help predict declination changes over time, ensuring your steering remains accurate throughout long-duration missions beyond our planet.
Inertial Guidance Units
While stellar reference systems provide the cosmic equivalent of latitude and longitude, Inertial Guidance Units (IGUs) serve as your spacecraft’s inner ear, constantly measuring orientation and acceleration without external references.
These sophisticated systems track your position by integrating accelerometer and gyroscope data over time, accounting for declination changes as you shift from Earth to space.
When operating an IGU, you’ll need to:
- Calibrate before launch to account for Earth’s magnetic declination effects
- Monitor drift during flight as the absence of true north references can lead to accumulated errors
- Apply correction algorithms that compensate for gravitational influences affecting your navigation
Though magnetic declination becomes less relevant in deep space, your IGU’s initial calibration remains essential during launch and re-entry when Earth’s magnetic field still influences your navigation systems.
Magnetometer Calibration Challenges
When you’re attempting to measure magnetic declination in the vacuum of space, you’ll face a unique set of calibration challenges that terrestrial navigation systems simply don’t encounter.
Your spacecraft’s magnetometer must account for its position relative to Earth’s magnetic field, requiring sophisticated models like the WMM for accurate predictions.
You’ll need precise calibration techniques that reference known points in space, comparing onboard readings against established magnetic field models to correct declination errors.
Even small inaccuracies in magnetic declination measurement can result in significant navigational discrepancies over extended journeys.
Don’t underestimate the importance of regular recalibration—Earth’s magnetic field constantly changes, requiring your spacecraft’s systems to update accordingly.
Without these adjustments, you’ll risk deviating from your planned trajectory as the magnetic declination values your navigation system relies on become increasingly outdated.
Compensating for Declination in Automated Navigation Systems
Because automated spacecraft must maintain precise trajectories across vast distances, proper compensation for magnetic declination remains fundamental to their guidance systems.
You’ll find that automated systems rely on the World Magnetic Model (WMM) to adjust calculations as the Earth’s magnetic field shifts over time.
When traversing through space, your spacecraft’s systems must:
- Integrate real-time magnetic field data to correctly align geographic coordinates with magnetic references
- Utilize combinations of magnetic sensors and gyroscopes to measure declination dynamically
- Apply continuous adjustments that prevent minor errors from becoming substantial trajectory deviations
This compensation process is particularly critical during long-duration missions where magnetic declination variations could otherwise compromise positioning accuracy.
Without these adjustments, even sophisticated guidance systems would gradually drift off course.
Declination Variations Across Different Planetary Bodies
Unlike Earth’s relatively predictable magnetic field, other planetary bodies in our solar system exhibit dramatically different declination patterns that directly impact direction-finding strategies.
You’ll encounter unique challenges when steering on Mars, where the weak magnetic field creates localized variations in magnetic declination across its surface.
The Moon presents a different scenario altogether—with no global magnetic field, compasses won’t work, eliminating declination concerns.
Jupiter’s extraordinarily powerful magnetic field (20,000 times stronger than Earth’s) creates extreme declination variations, especially within its radiation belts.
Venus, with its retrograde rotation, lacks a significant magnetic field, resulting in negligible magnetic declination.
When planning interplanetary missions, you must account for these distinct magnetic characteristics to plot accurate courses, as traditional Earth-based direction-finding assumptions simply won’t apply beyond our home planet.
Real-World Mission Examples of Declination Challenges
Space missions throughout history have grappled with declination challenges that drastically impacted their success or failure. When you examine these cases, the critical nature of magnetic declination becomes clear:
- Apollo navigators had to precisely account for magnetic declination during trajectory calculations—even minor errors could have caused significant lunar landing point deviations.
- Spirit and Opportunity Mars Rovers relied on magnetic compasses despite Mars’ varying declination patterns, which directly affected their ability to navigate the surface accurately.
- Both the ESA’s Rosetta mission to a comet and the ISS required continuous declination adjustments to maintain proper alignment and positioning.
Satellite operations further demonstrate this importance, as declination errors can compromise antenna alignment and potentially sever communications with Earth-based control centers.
Future Technologies for Precise Declination Determination
While current navigation systems have served space missions adequately, emerging technologies promise to revolutionize how we determine magnetic declination with unprecedented accuracy.
You’ll soon witness satellite navigation systems using atomic clocks that dramatically improve positional accuracy and magnetic declination calculations.
AI and machine learning algorithms will enhance predictive capabilities for declination changes, enabling real-time navigation adjustments.
Quantum sensors offer extraordinary magnetic field measurement precision, while autonomous spacecraft with advanced magnetometers will continuously monitor local conditions.
Perhaps most exciting is the international collaboration to create higher-resolution global magnetic field maps.
These detailed databases will greatly benefit both Earth and space navigation systems by providing more accurate magnetic declination data regardless of your location—whether you’re operating a rover on Mars or piloting a spacecraft beyond our solar system.
Declination Training for Space Navigation Professionals
Five core components form the foundation of effective declination training for space navigation professionals.
You’ll need thorough understanding of magnetic declination to align spacecraft systems with true geographic coordinates for precise trajectory calculations. Continuous education guarantees you can adapt to varying magnetic conditions encountered during missions.
Training programs typically emphasize:
Training programs empower space navigators with essential tools to handle Earth’s complex magnetic variations during critical missions.
- Proficiency with prediction models like the World Magnetic Model (WMM) to account for declination changes throughout mission duration
- Study of historical declination shifts to anticipate navigational challenges in different environments
- Hands-on experience with measurement techniques including declinometers and online resources
This training proves especially vital for long-duration missions where Earth’s magnetic field variations could greatly impact navigation accuracy if not properly accounted for in your calculations.
Frequently Asked Questions
Why Is Magnetic Declination Important for Navigation?
Magnetic declination is important for your navigation because it helps you adjust for the difference between magnetic and true north. Without this correction, you’ll accumulate significant positional errors as you travel longer distances.
What Is the Significance of Declination?
Declination’s significance lies in your ability to distinguish true north from magnetic north. You’ll need this knowledge to navigate accurately, as it’s essential for adjusting compasses and determining precise locations during your journeys.
What Is Declination in Navigation?
Declination in navigation is the angle between magnetic north and true north. You’ll need to adjust for this when using a compass to guarantee you’re accurately plotting your course during land travel.
How Does Declination Work in Astronomy?
In astronomy, declination works as a celestial latitude coordinate. You’ll use it with right ascension to pinpoint objects in the sky, measuring angular distance north (+) or south (-) from the celestial equator.
In Summary
You’ve seen how declination provides the critical north-south positioning that makes precise space navigation possible. Whether you’re plotting interplanetary trajectories or maintaining orbital parameters, you’ll find that even slight declination errors compound dramatically over cosmic distances. As you’ve learned, it’s not just academic—real missions succeed or fail based on these calculations. As technology advances, you’ll continue to rely on declination as a fundamental cornerstone of space exploration.
Leave a Reply